Table of Contents
Phosphorylation Definition
Phosphorylation is the transfer of a phosphoryl group from a donor to a receiver molecule.
Phosphorylation is a biological process that involves the addition of a phosphate molecule to an organic substance such as glucose or adenosine diphosphate (ADP).
In the latter case, the addition of the phosphate group to transforms ADP to adenosine triphosphate (ATP), a crucial molecule that provides energy for a variety of functions in living cells, including nerve impulse transmission and muscular contraction.
The addition of a phosphate molecule to an organic substance is the easy response.
What is Phosphorylation?
Phosphorylation is a crucial biological step in which phosphate molecules are added to an organic component to make it useful for a variety of activities in a live organism.
Another similar term is “phosphorylate,” which refers to the addition of a phosphoryl group to an organic molecule.
The phosphorylation reaction is very important in biology since it is required for numerous biological processes including as apoptosis, inflammation, metabolic control, subcellular transport, and proliferation.
The transfer of phosphate molecules to a protein is known as phosphorylation in biology. In a living creature, this transfer prepares proteins for specific functions.
In humans, phosphorylation takes place on the side chains of three amino acids: (1) tyrosine (an amino acid produced in the body of living creatures from another amino acid called “phenylalanine”), (2) serine, and (3) threonine. However, histidine phosphorylation has also been shown in certain investigations.
Tyrosine, serine, and threonine are the amino acids that can be phosphorylated.
Phosphorylation is a reversible process, which means it may add and remove phosphate molecules. Kinases are the enzymes that are responsible for adding phosphate groups to proteins. The enzymes that remove these phosphate groups are known as “phosphatases.”
Purpose of Phosphorylation
Phosphorylation plays a crucial role during a sort of biological processes. It plays a role in the cell’s control of protein activities and signal transmission. Cell growth, signal transmission, cell development, protein synthesis, and cell division are all aided by it.
transmission, cell development, protein synthesis, and cell division are all aided by it. The reaction of phosphorylation is reversible. Phosphorylation and dephosphorylation (opposite of phosphorylation) activate and deactivate the majority of enzymes and receptors, respectively. Phosphorylation of enzymes acts as an on/off switch, changing the activity or function of the enzyme.
The phosphate molecule is a critical component of biomolecules. The researchers discovered that a huge number of metabolites (metabolite intermediates or end products) are phosphorylated in cells, resulting in the production of phosphates.
Initially, two scientists, Thomas Rall and Earl Sutherland, were doing study on the link between glucagon and epinephrine and therefore the liver enzyme glycogen phosphorylase.
The hormones in question were discovered to be involved in the synthesis of 3,5-cyclic adenosine monophosphate. This chemical was discovered to function inside a cell to alter the activity of phosphorylase. As a result, 3,5-cyclic adenosine monophosphate is a molecule that acts as a messenger for hormonal signals.
The phosphorylation of tyrosine regulates protein activity. Tyrosine is an amino acid that our bodies make from phenylalanine, another amino acid. The phosphorylation of the regulating tyrosine causes enzymatic activity-related modifications in the substrate. Phosphorylation can also be used for the following objectives;
1. Protein dissection
2. Control of Enzyme Inhibition
3. Glycolysis activity
4. Protein-protein communication
5. Controls energy-intensive chemical processes.
The Mechanism of Phosphorylation
Protein phosphorylation takes place on the side chains of three different amino acids, notably serine, threonine, and tyrosine, which are phosphorylated reversibly in eukaryotic cells.
The terminal phosphate group is targeted by the nucleophilic (–OH) group in these amino acids. The nucleophilic (–OH) group in these amino acids binds to the phosphate terminal group (-PO32-) and is transferred from the phosphate group to the amino acid side chain by the usual phosphoryl donor adenosine triphosphate (ATP), commonly known as ATP phosphorylation.
Magnesium (Mg2+) facilitates this transition, which lowers the phosphoryl transmission threshold in the γ- and ß-phosphate groups through the nucleophilic (–OH) group.
Due to the large amount of free energy created when ATP is disrupted to generate adenosine diphosphate (ADP) in the phosphate-phosphate bond, this phosphorylation process is one-way.
Phosphorylation is a critical step in controlling protein function across a wide range of proteins and is strongly connected to protein activity. By causing conformational changes in the phosphorylated protein, phosphorylation regulates protein function and cell signalling.
All of these phosphorylation changes have a two-fold effect on the protein. To begin, conformational changes in the protein are used to monitor its catalytic activity. As a result, phosphorylation can either activate or deactivate a protein.
Second, phosphorylated proteins attract surrounding proteins with structurally preserved domains that recognise and connect to phosphomotifs. The amino acids that differentiate these domains are distinct.
Phosphotyrosine (pY) selectivity is demonstrated by the Src homology2 (SH2) and phosphotyrosine-binding (PTB) domains, however variations between these two kinds are unique to various phosphotyrosine species.
Phosphoserine (pS) is recognised by both the MH2 and WW domains, but phosphothreonine (phosphorylated threonine) (pT) is recognised by forkhead-associated FHA domains.
The ability of phosphoproteins to attract other proteins, in which phosphorylated signal protein is recruited downstream, is important for signal transduction.
Protein phosphorylation is one of the reversible post-translational modifications (PTM) that is controlled by kinases to phosphorylate and phosphatases to dephosphorylate substrates. The phosphorylated protein dynamics behaviour in a cell is promoted by both enzyme types.
In reality, the size of the phosphoproteome in a single cell is determined by the spatial and temporal equilibrium of kinase and phosphatase concentrations inside the cell, as well as the catalytic performance at a specific location.
Protein Kinases
Kinases are enzymes that help phosphate transfer to substrates. More than 500 kinases have been predicted from the human proteome. This group of proteins can be found in the human kinome.
Lipids, carbohydrates, nucleotides, and proteins are among the substrates for kinase action. While guanosine triphosphate is utilised in a few kinases, ATP is nearly universally used as a co-substrate in protein kinases.
ATP is ideal for the transition of α-, β– or γ- phosphate classes in nucleotidyl-, pyrophosphoryl-, and phosphoryl conversion. The ATP binding site is generally preserved, although kinase substrate selectivity varies.
Protein kinases are divided into subgroups based on their catalytic domains, which include tyrosine kinases and serine/threonine kinases. Serine / threonine kinases account for about 80% of the mammalian kinome, while phosphoproteomes pS and pT account for more than 90% of the phosphoproteome.
According to research, the relative abundance ratio of pS:pT:pY in a cell is 1800:200:1. Despite the fact that pY is not as common as pS and pT, global tyrosine phosphorylation is at the top of biomedical science’s priority list due to its link to human sickness via the dysregulation of receptor tyrosine kinases (RTKs).
The protein kinase substrate’s specificity is determined not only by the desired amino acid, but also by the consensus sequences that surround it. In certain kinases, these consensus sequences allow for the phosphorylation of a single protein as well as many phosphorylated substrates (>300).
As a result, kinases may be able to phosphorylate single or multiple amino acids in individual proteins if kinase-specific consensus sequences are available.
Control subunits in kinases operate as activating or auto-inhibiting domains with various regulatory substrates. Phosphorylation of these subunits is a common method of regulating kinase activity. Most protein kinases are dephosphorylated and inactive in their natural state. Only a small percentage of phosphorylated kinases are fundamentally inefficient or inactive because they are constitutively active.
Phosphorylation and dephosphorylation must be combined in some kinases, including Src, indicating that this proto-oncogene is tightly controlled. By regulating the spatial connection between kinases and upstream and downstream regulators, scaffolding and adaptor proteins can also influence kinase behaviour.
The activity of certain kinases can be determined by incubating immunoprecipitate with substrates of different kinases and ATP. Commercial instruments are available for this type of test, and they can accomplish colorimetric, radiometric, or fluorometric identification.
Although this type of test shows the activity of specific kinases, including kinase enrichment, it does not show the proteins that kinases alter or the influence of endogenous phosphatase activity.
Signal Transduction Cascades
Because protein phosphorylation is reversible, cells may easily adjust to intracellular or external stimuli, this type of PTM is appropriate for signal transmission. One or more proteins that sensitively represent the physical sensors by binding a ligand, cleavage, or some other response differentiate signal transduction cascades of the signal.
When kinases are phosphorylated, these receptors activate downstream, then phosphorylate and activate their cognate downstream substrates, as well as other kinases, before reaching the exact response.
Signal transduction pathways can be linear, with kinase A activating kinase B, which then activates kinase C, and so on. Kinases A trigger multiple kinases, which in turn excite more kinases, revealing signalling pathways that amplify the initial signal. With this type of communication, a single molecule can induce widespread cellular activity like proliferation, for example.
Protein Phosphotases
The pace and breadth of phosphorylation-related signalling are controlled by three pathways:
1. Separation of the stimulating ligand from the other ligands
2. Proteolysis of a substrate by a kinase
3. Phosphatase Dephosphorylation
Around 150 phosphatases of pS, pT, and pY residue types are known to be present in the human proteome. Although both phosphatase groups have the same ultimate goal of dephosphorylation, they go about it in distinct ways.
Serine/threonine phosphatases, bimetallic centres (Fe/Zn), and tyrosine control quick hydrolysis in the phosphorus group of the phosphorus atom. Phosphatases produce a thiophosphoryl covalent intermediate that permits tyrosine residues to be removed.
In living cells, hosphorylation is crucial since it is through this process that energy-rich molecules (such as ATPs) are produced. Phosphorylases and kinases, for example, catalyse the addition of a phosphoryl group (phosphate) to ADP, resulting in ATP.
Phosphorylation Types
Phosphorylation and dephosphorylation may occur in a variety of substances.
1. Glucose Phosphorylation
When glucose is phosphorylated with different other sugars during its catabolism, it is referred to as phosphorylation. D-glucose is first transformed to D-glucose-6-phosphate in glycolysis, for example. Glucose is a tiny molecule that penetrates quickly. Because glucose is so little, it gets into the cells fast.
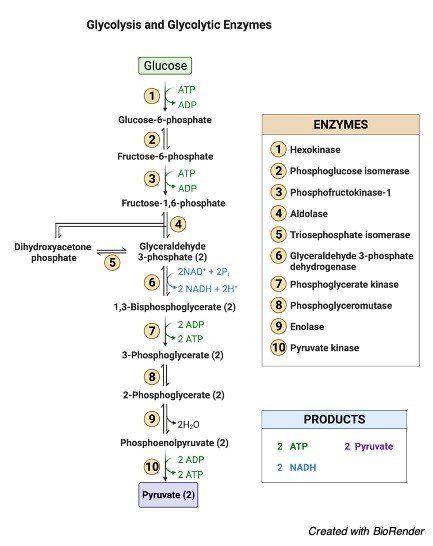
Phosphorylation is a larger enzyme that can’t get into the tissue rapidly. Phosphorylation is also necessary for the control of blood glucose levels. The synthesis of glycogen is directly proportional to the content of glucose. Phosphorylation of glucose is also linked to heart development.
2. Protein Phosphorylation
Phosphorylation of proteins occurs when an amino acid is linked to a phosphorylate group. Even though phosphorylation occurs with threonine and tyrosine in eukaryotes and histidine in prokaryotes, serine is the most common amino acid.
When one phosphate group interacts with the hydroxyl (-OH) group of a serine, threonine, or tyrosine side chain, this is referred to as an esterification process.
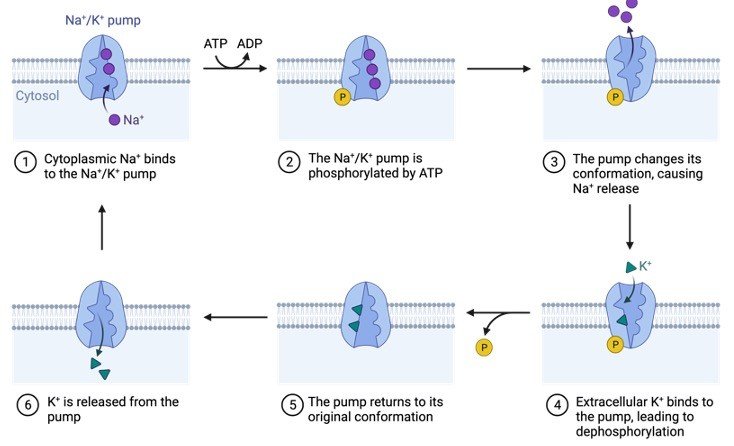
Protein kinase attaches the amino acid to the phosphate group in a covalent manner. The mechanism differs significantly between prokaryotes and eukaryotes.
The best-studied kind of phosphorylation appears to be post-translational modifications (PTM), which indicate that proteins are phosphorylated after conversion from an RNA sequence. Dephosphorylation and reversal processes were performed by protein phosphatases.
Protein phosphorylation may be seen in the phosphorylation of histones, which is a good example. In eukaryotes, DNA is linked with chromatin-forming histone proteins.
Histone phosphorylation alters the DNA-protein and protein-protein interactions, affecting chromatin shape. When DNA is degraded, phosphorylation occurs to provide new locations across damaged DNA so that repair procedures can operate correctly.
In addition to its importance in DNA repair, protein phosphorylation plays an important role in metabolism and signalling cascades.
3. Oxidative Phosphorylation
Oxidative phosphorylation is the process through which a cell releases and absorbs chemical energy. In the eukaryotic cell, reactions occur within the mitochondria. Interactions in the electron transport chain and chemiosmosis are involved in oxidative phosphorylation.
Finally, the transfer of electrons from molecules and proteins into the inner membrane of mitochondria via the electron transport chain produces the energy needed to generate adenosine triphosphate (ATP) in chemiosmosis.
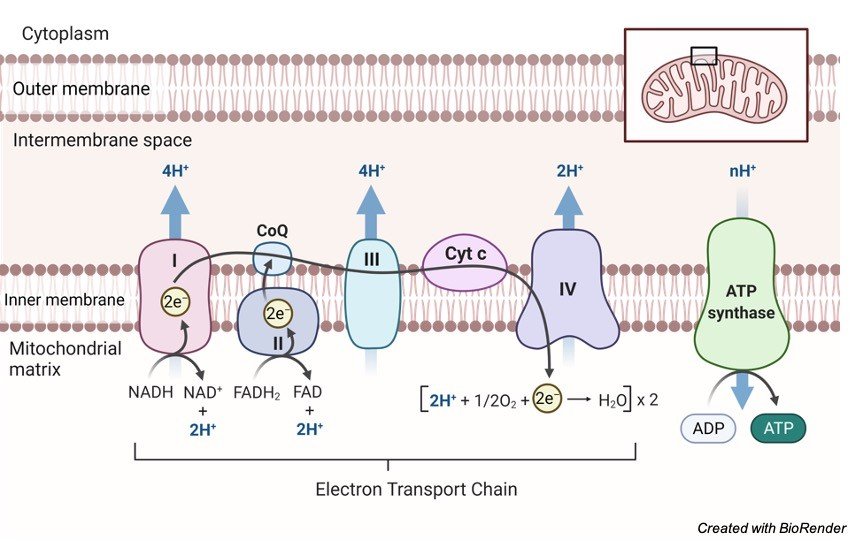
NADH and FADH2 send electrons to the electron transport chain during this procedure. As electrons go down the chain, they release energy as they go from higher to lower energy. Much of the energy used to create an electrochemical gradient is spent on transporting hydrogen ions (H+).
By interacting with H+ ions at the network’s end, electrons are transformed to oxygen and water is created. The ATP synthesiser receives ATP synthase energy from these ions.
As ATP is dephosphorylated, the phosphate group is degraded, producing energy that may be used by the cell. It’s possible that adenosine isn’t the sole base that gets phosphorylated to make AMP, ADP, and ATP. Guanosine, for example, has the potential to produce GMP, GDP, and GTP.
Detecting Phosphorylation
The phosphorylation of a molecule is detected using a mass spectrometer, electrophoresis, and antibodies. Phosphorylation sites, on the other hand, are difficult to identify and describe. Fluorescence, electrophoresis, and immunoassays are all employed in conjunction with isotope marking.
Biological Importance of Phosphorylation
Organic phosphates, which help living organisms develop tissues through anabolic chemical reactions, are important in the transfer of energy.
The importance of organic phosphates in metabolism was demonstrated and the findings were reported in Harden and Young’s study, which showed that when inorganic phosphorus was applied to the media and then converted to organic phosphate, the fermentation of glucose by cell-free yeast juice quickly improved.
Physiological Importance of Phosphorylation
Phosphoric acid is used to make the cell protoplasm. Phosphorylation is thus a crucial chemical activity for all cells. It’s also important for absorbing and metabolising a wide variety of foods.
The following is a list of its effects on several metabolic pathways:
In relation to Carbohydrates:
1. Phosphorylation aids carbohydrate absorption by the intestinal mucosa as well as glucose reabsorption by the kidneys. Hexose phosphate is produced in each of these epithelial cells. This molecule undergoes dephosphorylation, allowing hexoses to enter the circulation while leaving phosphoric acid alone.
2. Glycogen synthesis from glucose and glycogen breakdown into glucose are hypothesised to occur during phosphorylation in the liver and muscles.
3. Phosphorylation is common at all phases of chemical changes that occur after muscle contraction. Phosphorylation and dephosphorylation occur in this area in a cycle. Glycogen is broken down into lactic acid by a number of phosphorylated molecules.
In relation to Fats:
1. The absorbing epithelium produces neutral fats and phospholipids mostly during fat digestion. The enzyme phosphorylase is responsible for phosphorylation.
2. Phospholipids, particularly lecithin, are produced by the liver. The transfer of fat is an important stage. It also acts as the primary step of fatty acid oxidation. Fatty acid oxidation is a mitochondrial activity.
In relation to Proteins and Vitamins:
All phosphoproteins, including nucleoproteins, caseinogens, and others, must be produced. Phosphorylation is so expected to have a role in tissue oxidation, which is the process by which proteins, lipids, and carbohydrates are destroyed.
When it comes to the vitamin, it’s a no-brainer. Compounds like riboflavin phosphate, thiamine pyrophosphate, and others are found in certain vitamin B groups.
They are thought to be coenzymes in the oxidation and reduction phases of cell metabolism.
Regulation of Phosphorylation
The adrenal cortex is considered to be the source of the phosphorylation. Glucocorticoids increase the inhibition of phosphorylation after adrenalectomy.
Through its adrenocorticotropic hormone, the anterior pituitary may offer sophisticated phosphorylation control to the adrenal cortex.
The marasmic condition of adrenal cortex disorders may be explained in part by phosphorylation disturbance, which impairs absorption, metabolism, and body nutrition.
Phosphorylation Citations
Share